What's New Archive
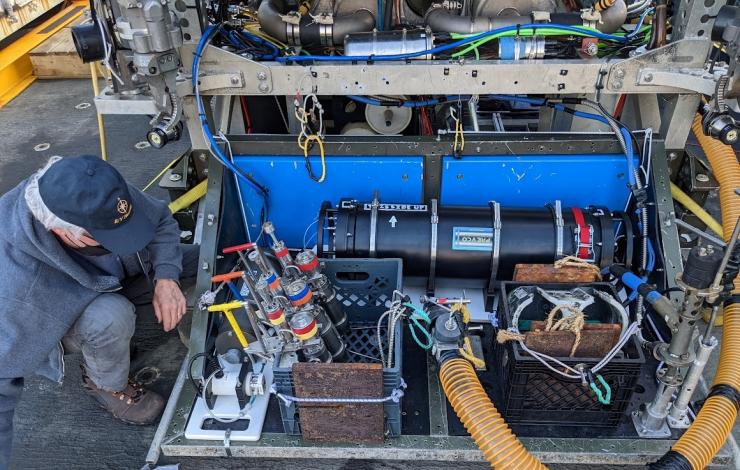
Dave Butterfield checking fluid connections to the methane spectrometer (large black pressure case) mounted at the back of ROV Jason’s tool sled. Other equipment used on this dive include the auxiliary pump at left, 3 titanium gas-tight sampler with colored T-handles, a suction sampler (large orange hose) and the Hydrothermal Fluid Sampler intake nozzle at far right. Photo Credit: Andrew Fahrland (OKSI)
NOAA PMEL, University of Washington and OptoKnowledge Systems, Inc. (OKSI) successfully conducted the first deep water test of a new methane analyzer to measure the concentration and carbon isotope ratio of methane near Axial Seamount. This new instrument can collect data that will improve scientists’ understanding of carbon cycling, greenhouse gasses, and sub-seafloor chemosynthetic reactions.
After 3 years of testing and development, the analyzer was mounted on the remotely operated vehicle Jason and coupled to PMEL’s hydrothermal fluid and particle sampler (HFS) during a recent cruise on the Research Vessel Thompson. The methane analyzer combines a novel laser absorption spectroscopy gas sensor with a membrane-free approach to water sampling. While the research cruise had to be cut short, the team successfully was able to collect valuable data and coordinate samples for cross-calibration during one ROV dive.
While collecting data with the methane analyzer, researchers simultaneously sampled the same fluid in gas-tight samplers attached to the fluid manifold and in custom samplers that are part of the HFS. Methane concentrations analyzed onboard the R/V Thompson by gas chromatography on fluid samples from HFS piston and bag samplers agree well with the in-situ methane concentrations determined by the analyzer.
Ultimately, the instrument worked well on the ROV and it is expected that the methane spectrometer will have many useful applications.
Learn more about this technology development and methane work on PMEL’s Earth Ocean Interactions research page.
Instrument Development:
The methane analyzer was developed by OKSI with initial funding from the NOAA Small Business Innovation Research (SBIR) Program. The SBIR Program, also known as America’s Seed Fund, awards seed funding to U.S. small businesses to support high-risk, high-reward research and development of new technologies. In 2016, OKSI received a NOAA SBIR Phase I award to develop an instrument that could analyze underwater gasses, specifically methane and its isotopes. The company was awarded follow-on Phase II funding in 2017.
The effort has since transformed into a Phase III collaboration with PMEL. OKSI and NOAA PMEL were funded in 2019 through a National Ocean Partnership Program (NOPP) grant with NOAA Ocean Exploration support to continue development of the methane spectrometer and deploy it in deep water. In 2021, PMEL and OKSI successfully completed shallow-water methane concentration measurements in Puget Sound. The Puget Sound test showed that the instrument can take measurements of methane concentration in-situ and report those measurements in real-time to an operator on board a ship with an update rate approximately every 6 minutes.
More About the Sensor:
For the new spectrometer instrument, it is important to verify that the in-situ measurements agree with established discrete sampling and analysis for methane concentration and carbon isotope ratio. The PMEL hydrothermal fluid sampler, first developed in 1998, will deliver warm hydrothermal fluids from diffuse vents with elevated methane concentrations to the methane spectrometer and simultaneously take coordinated discrete samples for laboratory analysis of concentration and carbon isotope ratio to cross-calibrate the in-situ measurements from the methane sampler. Tamara Baumberger is collecting samples in specialized gas-tight samplers for carbon isotope and methane concentration analysis.
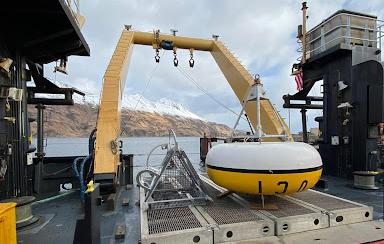
FOCI mooring ready for deployment in the Bering Sea aboard the NOAA Ship Oscar Dyson. The M2 surface mooring that has been deployed each spring in the southeastern Bering Sea for over 25 years. This critical mooring provides year-round measurements of temperature, salinity, nitrite, chlorophyll, and currents in this highly productive area. For the last 10 years, partial pressure in CO2 (pCO2) and pH measurements have also been taken at M2.
An annual survey is underway to provide baseline fisheries and oceanographic data to support sustainable management of living resources in the Bering Sea and the rapidly changing US Arctic ecosystem. These surveys provide key data in understanding and monitoring events such as sea-ice loss and the cold pool and how these are impacting the Arctic ecosystem.
This spring mooring cruise brings together scientists from NOAA’s PMEL and Alaska Fisheries Science Center, University of Washington, US Fish and Wildlife, and the University of Alaska. While aboard the NOAA Ship Oscar Dyson, the scientists will service a biophysical mooring array in the Bering Sea, collect conductivity, temperature, depth (CTD) profiles, zooplankton and ichthyoplankton samples and conduct special projects related to harmful algal blooms and zooplankton machine learning. Results from these observations and experiments will help describe important ecosystem linkages among climate, plankton, fishes, birds and mammals.
EcoFOCI will be field testing and using several technologies this summer, including pop-up floats, a remote access sampler and a new shallow-water glider. These technologies aim to enhance shipboard and mooring research with more data collection in a fine scale region.
NOAA’s EcoFOCI program is leading 5 research cruises this March to October in the Alaska region.
Find blog updates on the NOAA Alaska Fisheries Science Center webpage: https://www.fisheries.noaa.gov/region/alaska#science
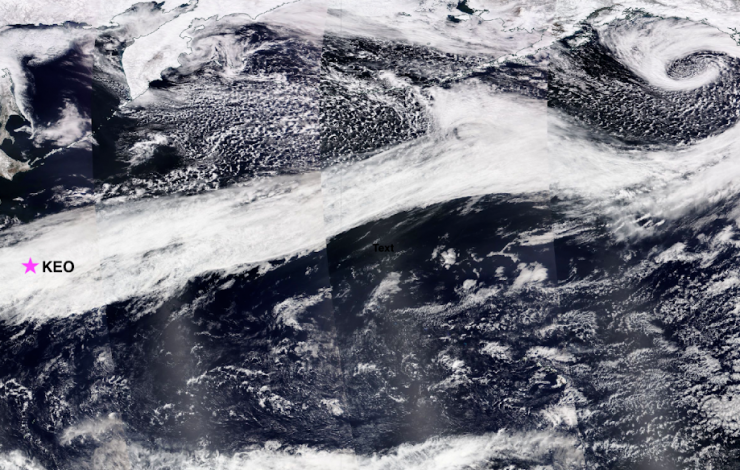
The KEO moored buoy (pink star) was deployed just in time to observe an intense atmospheric river (as shown in the satellite image) that connected the western Pacific to the east and brought stormy weather and heavy precipitation to the Pacific Northwest. Click on image to see full image. Cloud image created from worldview.earthdata.nasa.gov
The KEO Climate Station deployment aboard the R/V Kiayo Maru #1 took about 6 hours. Data from this key climate observation moored buoy can be used to help improve weather and storm forecasts, inform climate projections and verify satellite products and models. Photo Credit: Patrick Berk/University of Washington and NOAA PMEL
March 31, 2022: The Kuroshio Extension Observatory (KEO) moored buoy was successfully deployed by NOAA PMEL and partners, renewing an ongoing OceanSITES time series that began almost 18 years ago. First deployed in 2004, its time series was disrupted by the COVID pandemic. KEO collects vital data in the Kuroshio Extension recirculation gyre, a region known to affect the development of storms over the North Pacific before they reach the United States.
The deployment was just in time for KEO to capture the air-sea interaction process underneath an intense atmospheric river that connected the western Pacific to the east and brought stormy weather and heavy precipitation to the Pacific Northwest.
KEO carries sensors for NOAA PMEL and partners from Japan and US academic institutions that measure surface air-sea heat and momentum fluxes, along with various oceanographic variables in the upper 525 meters and near the seafloor. New this year, the KEO-2022 mooring includes dissolved oxygen sensors, a nitrate sensor, and a testbed for new biodegradable “plastic-alternative” materials from the Japan Agency for Marine-Earth Science and Technology (JAMSTEC), the University of Washington-Applied Physics Lab’s bubble and acoustic observations, and a moored CO2 system.
KEO will also play a critical role in the Japanese national program “Middle-latitude atmosphere-ocean interaction hotspot in a changing climate system” (HotSpot-2), as an ocean observatory by providing a reliable platform for new sensors and the long-term, large-scale context of intensive field campaign observations.
Learn more about the research part of the Ocean Climate Stations program: https://www.pmel.noaa.gov/ocs/KEO
This work is funded by NOAA’s Global Ocean Monitoring and Observing Program to sustain in situ global ocean observations and information in support of research, monitoring, and prediction.
A new video highlighting PMEL's acoustics research and eDNA innovations to study Blue Whales off the coast of Newport, Oregon has been released in the “PMEL at Work” YouTube series.
This video features PMEL’s Acoustic Program’s Bob Dziak and Angela Sremba discussing ongoing research to develop technology to better understand blue whales by simultaneously collecting environmental DNA (eDNA) samples and recording acoustic calls through deploying drifting buoys with underwater hydrophones.
Over the summer of 2021, PMEL and OSU CIMERS scientists field tested three passive-acoustic hydrophones to identify and locate blue and fin whales. These new smart hydrophones have an internal microprocessor that communicates the location of the buoys to shore. PMEL developed software for the microprocessor that will analyze the hydrophone data using a blue whale call detection algorithm. The goal for this algorithm is to register a positive blue call detection and transmit this positive detection information, as well as the position of the buoy, via satellite communication to shore to let scientists know when and where a blue whale call is recorded. When a call is detected on multiple buoys, the shore based scientists can then determine the location of the calling whale, and then inform the ship’s crew that a blue whale is nearby. The location of the whale will be correlated with the results from collected eDNA samples.
This past summer was a successful field test of the hydrophone buoys in anticipation for upcoming fieldwork in the summer of 2022 where PMEL will simultaneously collect acoustic recordings and eDNA samples of blue whales off the Oregon coast. This project will improve interpretation of eDNA results by using a time-series sampling method combined with acoustic detection for tracking and localizing highly migratory whale species in remote open ocean habitats.
This is a collaborative project between Oregon State University Marine Mammal Institute and Cooperative Institute for Marine Ecosystem and Resources Studies (CIMERS) and NOAA.
The PMEL at Work video series highlights ongoing research activities and projects supporting NOAA’s mission to understand changes in the global ocean and its impact on climate, weather and ecosystems.
February 1 - March 7: NOAA PMEL scientists join NOAA Fisheries and an international team of researchers aboard the NOAA R/V Shimada to provide expertise in physical oceanography and lead hydrographic data collection, nutrient sampling, analysis and processing. EcoFOCI is taking part in the International Year of the Salmon expedition to help detect and monitor changes both within Pacific salmon and their respective ecosystems, especially in the Gulf of Alaska.
Pacific salmon are a uniquely important resource for countries across the North Pacific yet there are major scientific gaps in our understanding of the ocean phase of the salmon life cycle. This cruise will collect vital data to improve that understanding and aid in forecasting and management of salmon.
The expedition will include as many as five research vessels to conduct the largest ever pan-Pacific, epipelagic ecosystem survey during winter, focused on understanding salmon and their ecosystems. The 2022 Expedition will involve a full ecosystem survey with pelagic trawling and detailed sampling of marine life in the upper ocean and will include research on physical, biological and chemical oceanography. Novel technologies such as gliders, environmental DNA and genetic stock identification will be used to enhance research efforts. This collaborative international effort spanning the entire North Pacific includes scientists from Canada, Japan, the Republic of Korea, the Russian Federation, and the United States.
The data collected by PMEL will provide key baseline and comparative data to the overall pan-Pacific collaboration focused on salmon recovery. During the expedition, PMEL will collect samples from over 30 CTD stations, deploy satellite-tracked drifters and several Argo floats, and collect carbon dioxide, nutrient and salinity measurements from an on-board flow-through system.
The research cruise will extend from 1 February to 7 March in the central to western Gulf of Alaska. More about the expedition: https://yearofthesalmon.org/2022expedition/
Follow along with the expedition on the blog and more details online: https://www.fisheries.noaa.gov/west-coast/2022-pan-pacific-winter-high-seas-expedition
On January 15, 2022, the Hunga Tonga-Hunga Ha’apai volcano erupted off the coast of Tonga in the South Pacific Ocean, generating a tsunami and triggering tsunami alerts around the world. Most tsunamis are commonly caused by earthquakes and only about 5% of tsunamis are generated from volcanic activity (ITIC), making this a rare event captured by NOAA’s observing instruments.
Buoys and Saildrone uncrewed surface vehicles additionally recorded an air pressure wave associated from the eruption. The pressure wave from the volcano explosion was detected as far as the Mediterranean Sea and traveled about 312 meters/second (697 miles per hour) and circled the Earth three times before dissipating. The Krakatau eruption in 1883 was the last event of such scale. Krakatau produced similar air pressure waves and a devastating tsunami that claimed the lives of ~36,000 people and the destruction of hundreds of coastal towns and villages.
Deep-ocean Assessment and Reporting of Tsunamis (DART) systems are strategically deployed by NOAA and international partners around the Pacific Ocean to detect tsunami waves and send data in realtime to tsunami warning centers. These systems recorded the propagating tsunami across the Pacific and prompted expansion of the tsunami alerts for many coastlines in the Pacific. Those warnings may have saved lives at many coastlines that were later flooded by the waves, some as far as the Pacific coast of Peru.
Along with the tsunami wave amplitudes measured by the DART system, the atmospheric pressure wave associated with a shock-wave emanating from the volcano explosion was measured. The air pressure signal detected by weather station buoys is the leading signature before the tsunami wave train and may provide clues for the mechanism of this unusual tsunami generation. However, given that the pressure signal mixed with the tsunami amplitudes in the data, high-resolution air pressure measurements are needed to decipher the DART tsunami records.
Coincidentally, two NOAA-Saildrone drones were approximately 3500 nautical miles (~4028 miles) away from the eruption, the distance to drive between Anchorage, Alaska and Miami, Florida, in the eastern tropical Pacific Ocean. The two drones are part of a 6-month, ongoing Tropical Pacific Observing System (TPOS) mission targeting the eastern tropical Pacific hurricane genesis region and El Niño Southern Oscillation (ENSO) development. The two drones were able to detect an atmospheric pressure jump in high-resolution measurements, capturing crucial information associated with the remote volcanic activity in an observationally-sparse region of the ocean for post-analysis with the DART tsunami records.
The phenomena recorded in the data is unique and additional research and development is needed to accommodate these types of tsunami events in the model used to forecast tsunami events. PMEL tsunami researchers are analyzing the data from the various platforms to get a better understanding of this rare event.
PMEL has also previously studied the dynamics of a smaller scale eruptive activity of this volcano using acoustic data and will uncover and analyze additional acoustic data from hydrophones deployed across the Pacific when they are recovered later this year.
Read more on the TPOS 2021 Saildrone Mission on the blog page.
More about the event can be found on the PMEL Tsunami Research group events page.
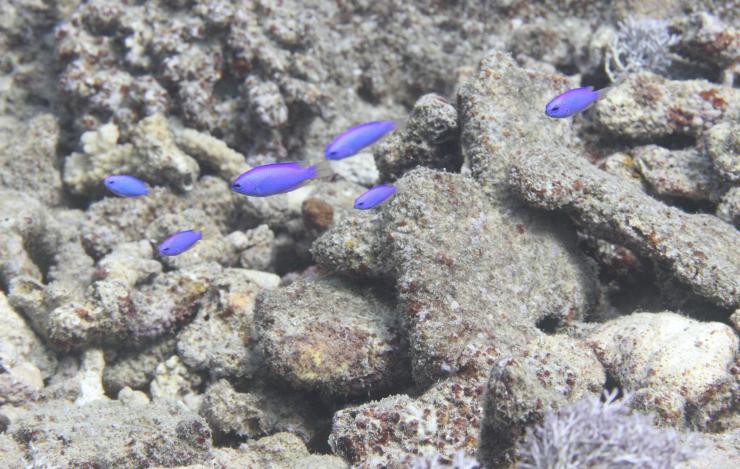
Damselfish in a tropical reef region within the National Park of American Samoa. Photo Credit National Park System. Click here to listen to a recording of damselfish.
A research team led by NOAA’s Cooperative Institute for Marine Ecosystem and Resource Studies at Oregon State University has developed an automated method that can accurately identify calls from a family of fishes.
The method takes advantage of data collected by underwater microphones known as hydrophones and provides an efficient and inexpensive way to understand changes in the marine environment due to climate change and other human-caused influences, said researchers from Oregon State’s Cooperative Institute for Marine Ecosystem and Resource Studies.
The findings were published in the journal Marine Ecology Progress Series.
Hydrophones are increasingly being deployed in the world’s oceans. They offer advantages over other types of monitoring because they work at night, in low-visibility conditions and over long periods of time. But techniques to efficiently analyze data from hydrophones are not well developed.
This new research led by Jill Munger when she was an undergraduate student, begins to change that. Munger came to Oregon State having worked more than 20 years in the corporate world. An avid scuba diver, she wanted to study the ocean. She received a fellowship from CIMERS to research underwater acoustics with Joe Haxel, who at the time was at the Hatfield Marine Science Center in Newport working with National Oceanic and Atmospheric Administration’s Pacific Marine Environmental Lab’s acoustic program.
Haxel handed her a hard drive with 18,000 hours of acoustic data collected over 39 months in a tropical reef region within the National Park of American Samoa. American Samoa is a U.S. territory in the western Pacific Ocean.
The data was collected via a 12-station hydrophone array maintained by NOAA and the National Park Service that is distributed throughout the world in water controlled by the United States. The hydrophones were designed and built by NOAA and CIMERS researchers at Hatfield Marine Science Center.
Munger decided to focus on calls from damselfish, in part, because they are distinctive. They grind their teeth to create pops, clicks and chirps associated with aggressive behavior and nest defense. She compared the sound to purring kittens. Quickly, it became apparent to her that manually listening to the recordings was not going to work.
“This is such a slow and tedious process,” she remembered thinking. “I have all this data, and I am just looking at a tiny, tiny portion of it. What’s happening in all the other parts that I haven’t had a chance to listen to?”
A conversation with her brother, Daniel Herrera, a machine learning engineer, sparked an idea. Could they use machine learning to automate the analysis of the data?
Machine learning algorithms build a model based on sample data, known as training data, to make predictions or decisions without being explicitly programmed to do so.
Machine learning techniques have been used to automate processing of large amounts of data from passive acoustic monitoring devices that collected sound data from birds, bats and marine mammals. The techniques have been used for fish calls, but it is an underdeveloped area of science, Munger said.
In this case, the machine learning sample or training data was 400 to 500 damselfish calls Munger identified by manually listening to the hydrophone recordings. With that start, Herrera, a co-author of the paper, built a machine learning model that accurately identified 94% of damselfish calls.
“We built a machine learning model on a relatively small set of training data and then applied it to an enormous set of data,” Munger said. “The implications for monitoring the environment are huge.”
Munger, who now works in the lab of Scott Heppell, an associate professor in Oregon State’s Department of Fisheries, Wildlife, and Conservation Sciences in the College of Agricultural Sciences, believes machine learning will increasingly be used by scientists to monitor many species of fish in the ocean because it requires relatively little effort.
“The benefit to observing fish calls over a long period of time is that we can start to understand how it’s related to changing ocean conditions, which influence our nation’s living marine resources,” Munger said. “For example, damselfish call abundance can be an indicator of coral reef health.”
Munger received input from National Park Service staff on the biology of the damselfish and the reef habitats in close proximity to the hydrophone.
Other co-authors of the paper are Haxel, Heppell, and Samara Haver, all of Oregon State; Lynn Waterhouse, formerly of John G. Shedd Aquarium in Chicago; Megan McKenna, formerly of Natural Sounds and Night Skies Division, National Park Service, Fort Collins, Colorado; and Jason Gedamke and Robert Dziak of NOAA’s Office of Science and Technology, Silver Spring, Maryland, and Pacific Marine Environmental Laboratory, Newport, respectively.
The following article was written by Sean Nealon at Oregon State University and adapted for NOAA PMEL. The Oregon State University news release is available online.
NOAA’s 2021 Arctic Report Card, released today at the American Geophysical Union’s Fall Meeting, documents the numerous ways that climate change continues to fundamentally alter this once reliably-frozen region, as increasing heat and the loss of ice drive its transformation into a warmer, less frozen, and more uncertain future.
The Arctic continues to warm more than twice as fast as the rest of the globe. The October-December 2020 period was the warmest Arctic autumn on record dating back to 1900. The average surface air temperature over the Arctic this past year (October 2020-September 2021) was the 7th warmest on record.
The total extent of sea ice in September 2021 was the 12th lowest on record. All 15 of the lowest minimum extents have occurred in the last 15 years. The substantial decline in Arctic ice extent since 1979 is one of the most iconic indicators of climate change.
Some of the fastest rates of ocean acidification around the world have been observed in the Arctic Ocean. Two recent studies indicate a high occurrence of severe dissolution of shells in natural populations of sea snails, an important forage species, in the Bering Sea and Amundsen Gulf.
The Arctic Report Card is an original, peer-reviewed environmental observations and analysis that documents rapid and dramatic shifts in weather, climate, terrestrial and oceanic conditions in the circumpolar region. This year’s report was compiled by 111 scientists from 12 nations. PMEL and UW Cooperative Institute scientists contributed to sections on surface air temperature (Dr. James Overland, Dr. Muyin Wang), sea ice (Dr. Kevin Wood) and on ocean acidification (Dr. Jessica Cross, Dr. Darren Pilcher).
Check out the visual highlights and more details of the full Arctic Report Card.
Read the original NOAA Press Release and the recorded AGU Press Conference.
New Puget Sound Marine Waters 2020 Report Released: Automated buoys and volunteers helped gather critical Puget Sound data during pandemic. Photo Credit: Puget Sound Partnership 2021
New Report Released: Automated buoys and volunteers helped gather critical Puget Sound data during pandemic
On October 27, the Puget Sound Marine Waters Work Group of the Puget Sound Ecosystem Monitoring Program released the tenth annual report on marine water conditions in Puget Sound providing a comprehensive long-term view and current assessment of the Puget Sound marine ecosystem. There were few extreme weather or ecological events in 2020, but overall, conditions in Puget Sound were generally warmer, sunnier, and wetter than in typical years. The report further reveals patterns and trends in numerous environmental parameters, including plankton, water quality, climate, and marine life. The observations in this report collectively provide both a comprehensive long-term view and current assessment of the Puget Sound marine ecosystem.
In Puget Sound, ocean acidification (OA) continues as does our understanding of patterns. Annual average atmospheric carbon dioxide (CO2) values over Hood Canal were high relative to globally averaged marine surface air, yet were at the same level as in 2019. OA in Puget Sound is of particular concern as estuarine processes, both natural and human-mediated, can also increase the CO2 content and lower the pH of marine waters. Moreover, coastal upwelling brings deeper waters with naturally higher CO2 concentrations upwards and into Puget Sound via the Strait of Juan de Fuca. Thus, Puget Sound is influenced by a variety of drivers that exacerbate the growing OA signal, making our waters particularly sensitive to these conditions. All of these changes have ramifications for marine food webs and are areas of active current research, including PMEL's Moored Autonomous pCO2 (MAPCO2TM) system collecting on atmospheric and surface seawater xCO2 (mole fraction of CO2) at the Ćháʔba· mooring off of La Push, WA and at the Cape Elizabeth mooring.
Having high-quality observations of carbon in the coastal environment is important for understanding coastal ocean carbon and its impact throughout the water column and the ecosystem. Learn more about PMEL's Carbon and Ocean Acidification Research.
Read the full report here.
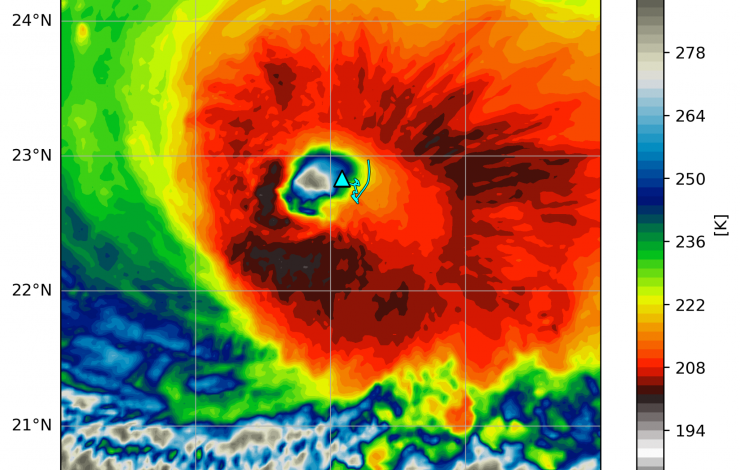
Saildrone 1045 location (triangle) overlaid on an infrared image from NOAA geostationary satellite (colors) at 16:00 UTC September 30, 2021. The saildrone track (light blue line) starts at 04:00 UTC on the same day. Courtesy of Edorado Mazza/University of Washington.
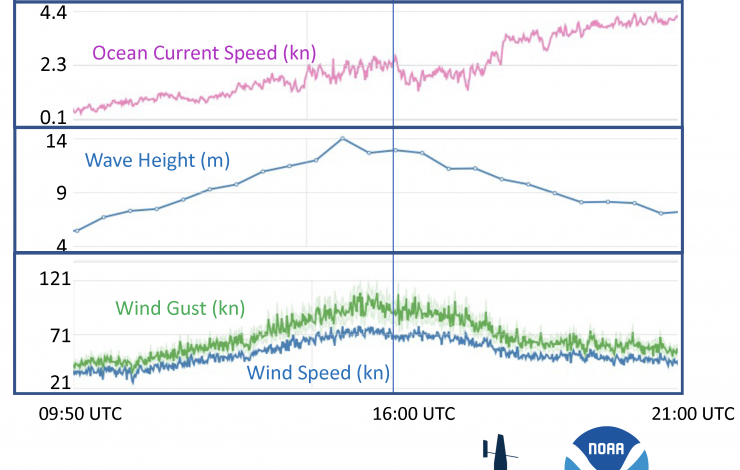
Time series of saildrone measurements of wind, humidity, pressure, current speed, wave heights and solar radiation on September 30, 2021. Vertical line marks the time when the saildrone was located inside the eyewall of Hurricane Sam. Click on image to view all the variables.
Saildrone 1045, one of the five drones PMEL and AOML deployed in the western Atlantic was steered into position to intercept Category 4 Hurricane Sam on September 30, 2021 to record critical data. The encounter required precise interpretation of hurricane forecasts and tight collaboration with Saildrone pilots. The saildrone measured one-minute sustained wind speeds of up to 90.6 miles per hour (40.5 m/s) with wind gusts up to 126.5 mph (56.5 meters/second), waves exceeding 46.4 feet (14.2 meters), and saturated air near the surface. The saildrone was in the hurricane eye next to the eyewall at 16:00 UTC / 12:00 EDT and was in sustained hurricane-force winds (at least 75 mph) for about two hours straight. The first ever drone camera images and video show a large amount of sea spray from the ocean surface and are a frightening display of the power of tropical cyclones.
This is the first time that sea surface conditions near a hurricane eye were observed by an uncrewed surface vehicle. Its success is built upon years of partnership between NOAA and Saildrone, Inc. with testing in progressively more hostile environments and 5 years of innovative engineering on the platform, sensors, and data processing, months of preparation leading to the deployment, and dedicated efforts of operating saildrones by the NOAA science team and Saildrone pilots during the deployment. This animation shows how the saildrone intercepted Hurricane Sam, and this animation, created by scientists at NOAA AOML, shows how the saildrone was positioned to intercept Hurricane Sam alongside saildrone observations of Hurricane Sam.
Prior to hurricane Sam, the NOAA saildrones also measured tropical storms Peter (9/21/2021), Henri (8/20/2021), Grace (8/17/2021) and Fred (8/13/2021). Read more about these encounters on the mission blog: https://www.pmel.noaa.gov/saildrone-hurricane2021/mission_blog.html
Read more on NOAA.gov: A world first: Ocean drone captures video from inside a hurricane